Research Overview
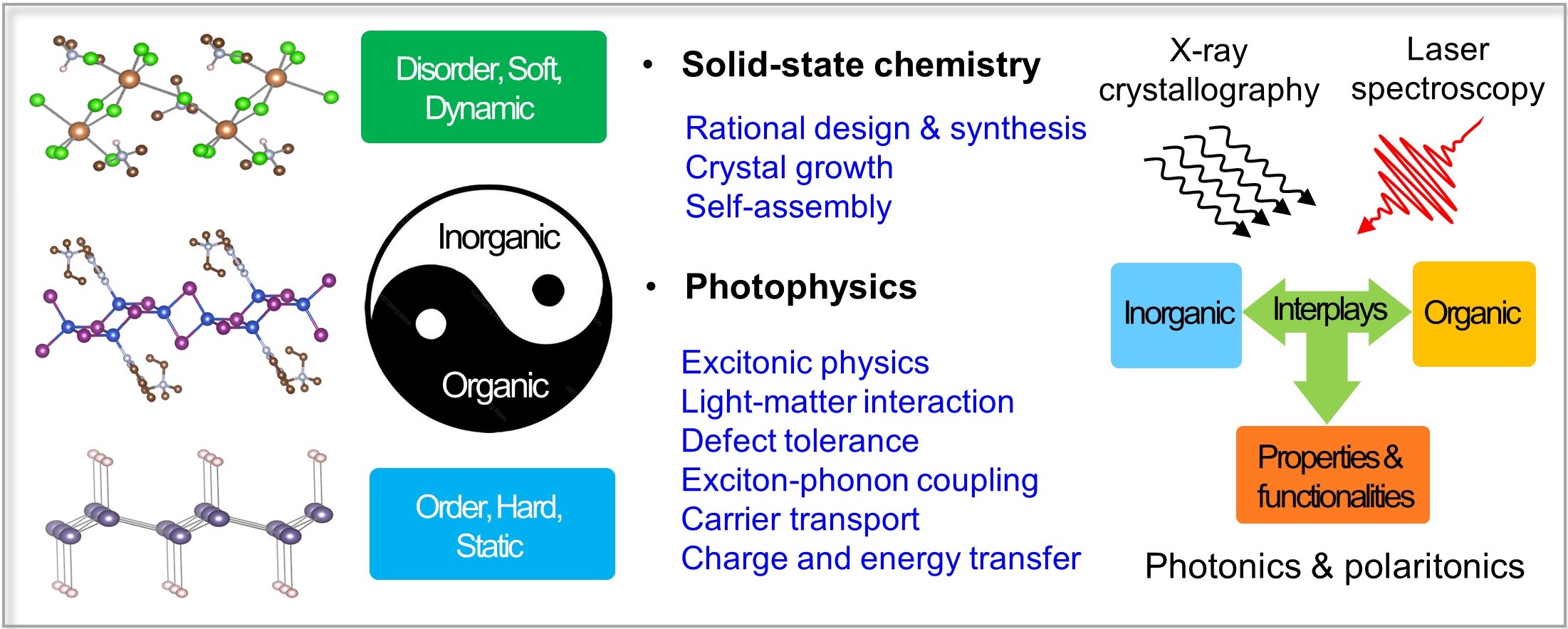
Our research is centered on the solid-state chemistry and photophysics of hybrid materials. Organic lattices are known to be disordered, dynamic, and soft, while inorganic lattices are often ordered, static, and hard. The conflict and balance between these opposites at the hybrid interfaces lead to unique solid-state chemistry and physics. A remarkable example is lead halide hybrid perovskites which have recently transformed photovoltaic and light-emitting research, and there are still many other hybrid systems to be explored. Our group develop new hybrid materials through molecular engineering and investigate the fundamental structure-property relationships. These materials exhibit advantageous properties and new functionalities not only due to the dimensional reduction but also the interaction at the hybrid interface. By synergistically integrating of our combination of expertise in both solid-state chemistry and optical spectroscopy, we aim to understand the chemical and electronic interplays between the organic and inorganic components and how these interplays translate to functional properties. We are interested in studying excitonic physics and strong light-matter interactions in hybrid materials and applying the understanding in application such as photonics, polaritonics, and quantum materials.
Research Projects
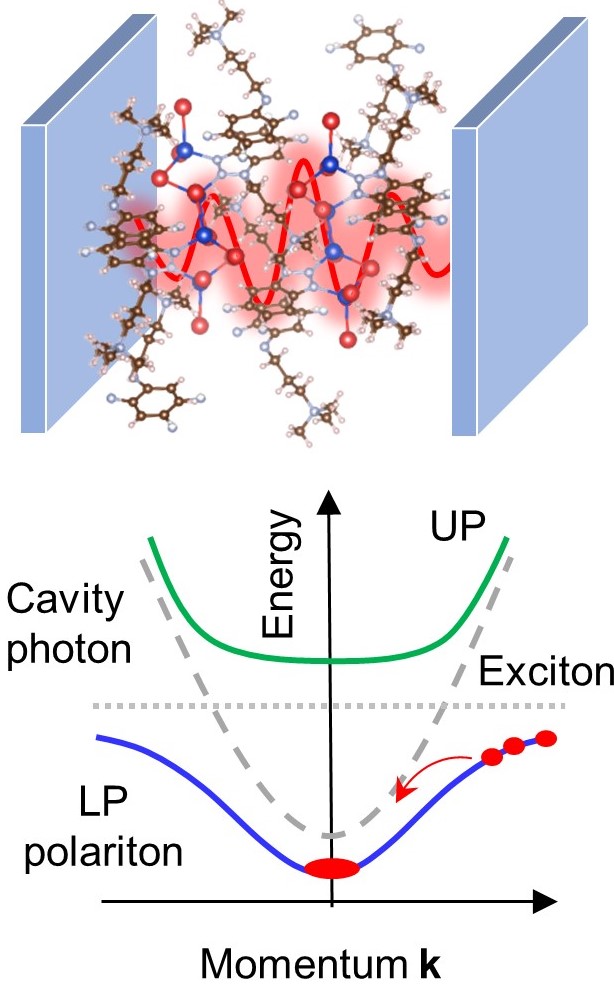
Light-matter interactions in hybrid materials.
We are interested in controlling excitons not only by chemical approaches, but also through coupling strongly to light. Strong coupling between excitons and cavity photons can result in the formation of exciton-polaritons, which are of part light and part matter character. As bosonic particles, exciton-polaritons can undergo Bose-Einstein condensation, akin to ultracold bosonic atoms. The low effective mass ~10−5 me and the corresponding long de Broglie wavelength of exciton-polaritons makes them exciting platforms to achieve Bose-Einstein condensates at high temperatures. The emerging hybrid materials provide unprecedented opportunities for studies of exciton-polariton phenomena due to the strong Coulomb interaction, e.g., the excitons can survive up to room temperature and at high carrier densities. We explore new hybrid materials and develop innovative strategies to fabricate high-quality optical microcavities, with the goal of realizing exciton-polariton condensation and superfluidity at room temperature. We study the nonlinear interactions of exciton-polaritons in hybrid semiconductor microcavities and explore them in quantum simulation for many-body physics and topological phases.
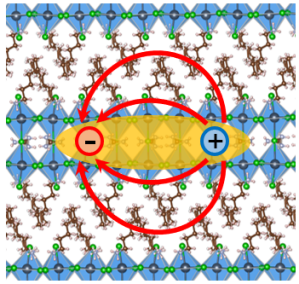
Excitons in hybrid quantum wells (QWs)
An exciton is a quasiparticle comprised of an electron and a hole bound by the Coulomb force, akin to a hydrogen atom. Excitons are fundamental to semiconductors and govern a range of optoelectronic processes. We aim to understand and control excitons in a class of self-assembled organic-inorganic QWs, where the inorganic layers act as potential wells and the organic layers act as potential barriers. Compared to III–V semiconductor QWs, the exciton binding energy can be increased by orders of miniguide, e.g., up to a few hundred meV, due to the reduced dielectric screening and quantum confinement. The substantially reduced Coulomb interaction in hybrid QWs offers rich physics related to excitonic complexes (e.g., biexciton and trion) and excitonic phase transitions, and photonic applications to be explored over a wide range of carrier densities at high temperatures.
The exciton properties are also strongly influenced by electron-phonon interactions, leading to intriguing optical properties, recombination dynamics, and transport behaviors. Unique to the hybrid QWs, these structures allow systematic tuning of the phonon by the thickness of the inorganic layer and by chemical identities of the organic molecules. We apply chemical synthesis to tune the dielectric and quantum confinement at the molecular level and use time- and space-resolved optical techniques to study the excitonic physics in hybrid QWs. Through these studies, we also aim to uncover the structural motif responsible for the exceptional carrier properties in halide perovskites, and to develop the design of principle for efficient and defect tolerant hybrid semiconductors.
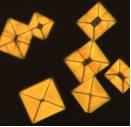
Rational design and synthesis of hybrid materials.
The vast number of organic molecules and inorganic components offer unlimited hybrid configurations for exploration. Our synthetic strategies mainly include designing functional organic molecules that are chiral, photosensitive, or conjugated, and selecting inorganic components that exhibit desirable optical, electronic, and magnetic properties. The organic molecules can also provide chemical reactivity to be explored. By varying the length or size of the organic molecules, the inorganic framework can consist of 0D, 1D, 2D or 3D network of corner-sharing, edge-sharing, or face-sharing inorganic polyhedra, giving rise to distinct functionality. The organic-inorganic interface plays a key role in determining the material property and functionality. For example, by controlling the relative energy levels between the inorganic framework and the organic molecules, band alignment of the hybrid interface can be engineered, which allow for controlling the flow of charge and energy at the interface. We are also interested in understanding the crystal growth of hybrid materials, with the goal of precisely controlling the self-assembly at the interface. We apply solution phase crystal growth, chemical vapor deposition and transport, and mechanochemical synthesis.